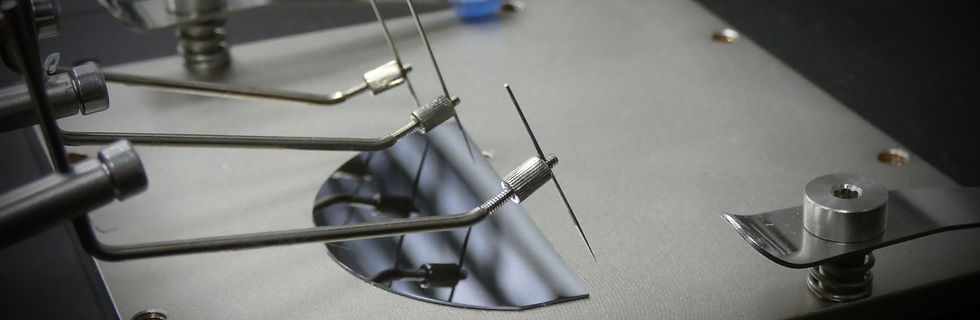
Research
Ratchet Based Ion Pumps
All Electric Ion Pumps for Water Desalination
Ion pumps are devices that use external power to introduce a net ionic flux. For example, in living cell membranes, nano scale channels pump different types of ions against a concentration gradient consuming energy from the hydrolysis of ATP. Although widely used in nature, there are very few technologies that can unleash the vast potential of ion pumps. Unlike electrons in electronic devices where contacts serve as nearly ideal sources and sinks for charge carriers, ions are sourced and removed by chemical reactions. However, since redox reactions have a threshold voltage that is defined by their Gibbs free energy and overpotentials, ion pumps that are based on such reactions are in most cases inefficient.
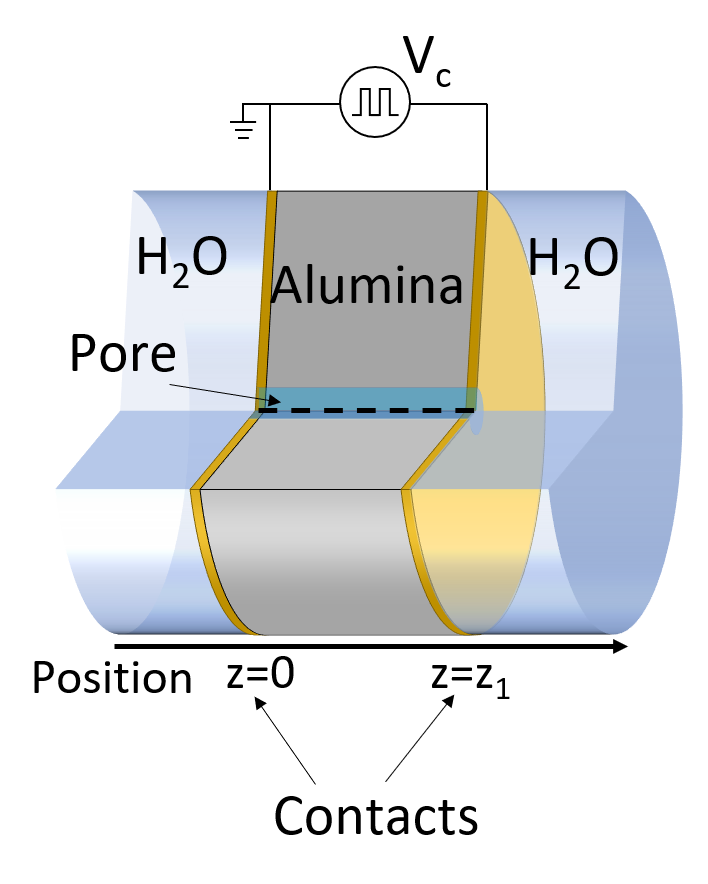
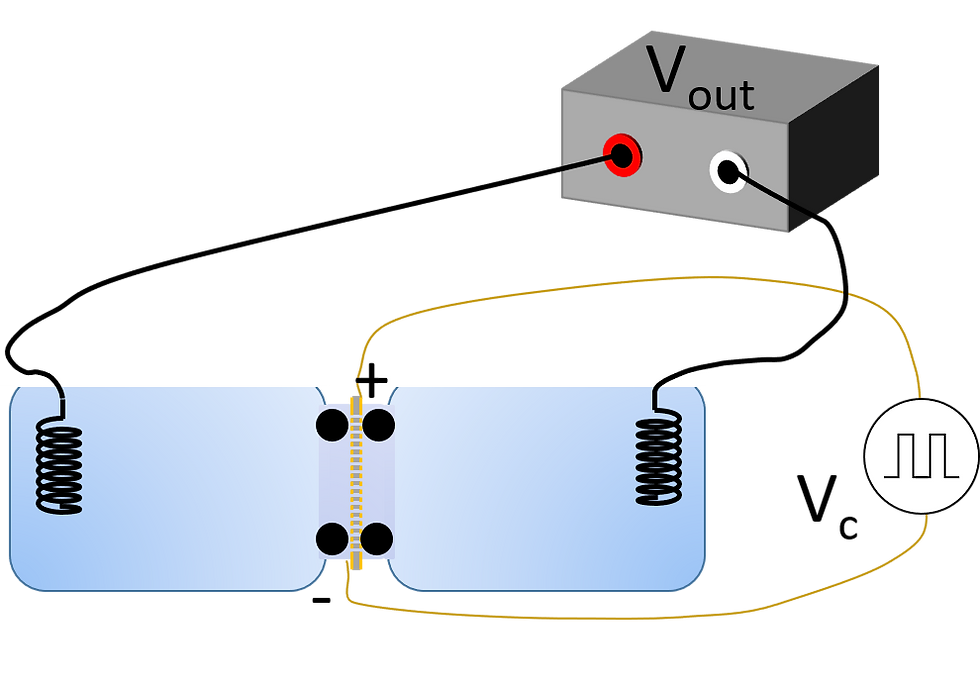
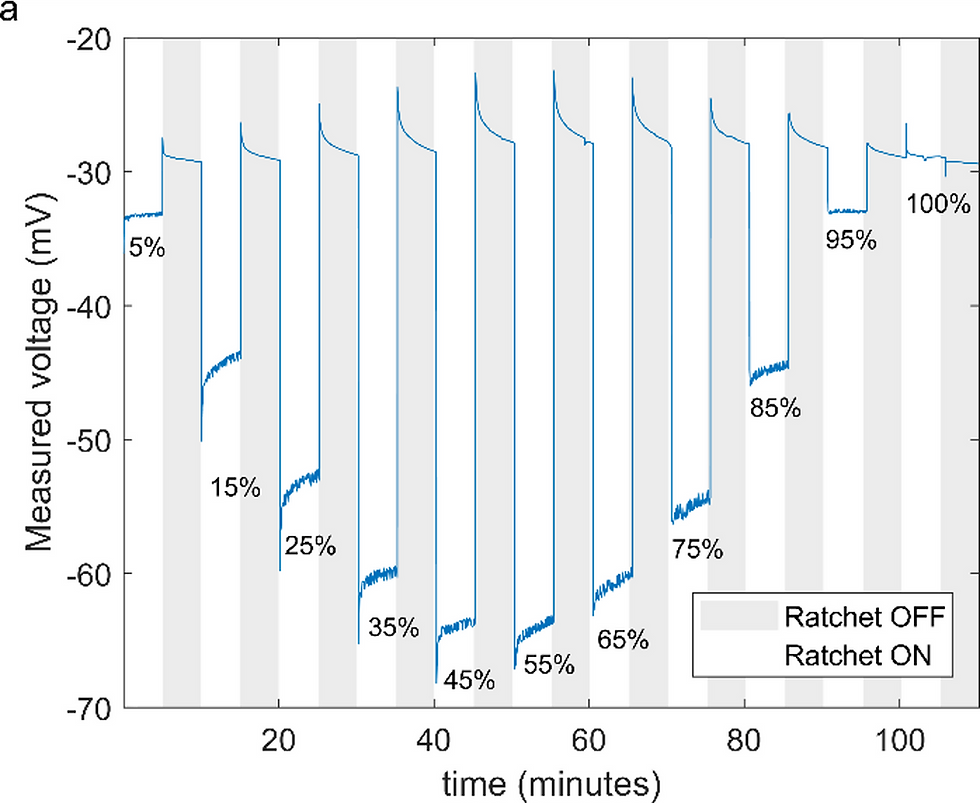
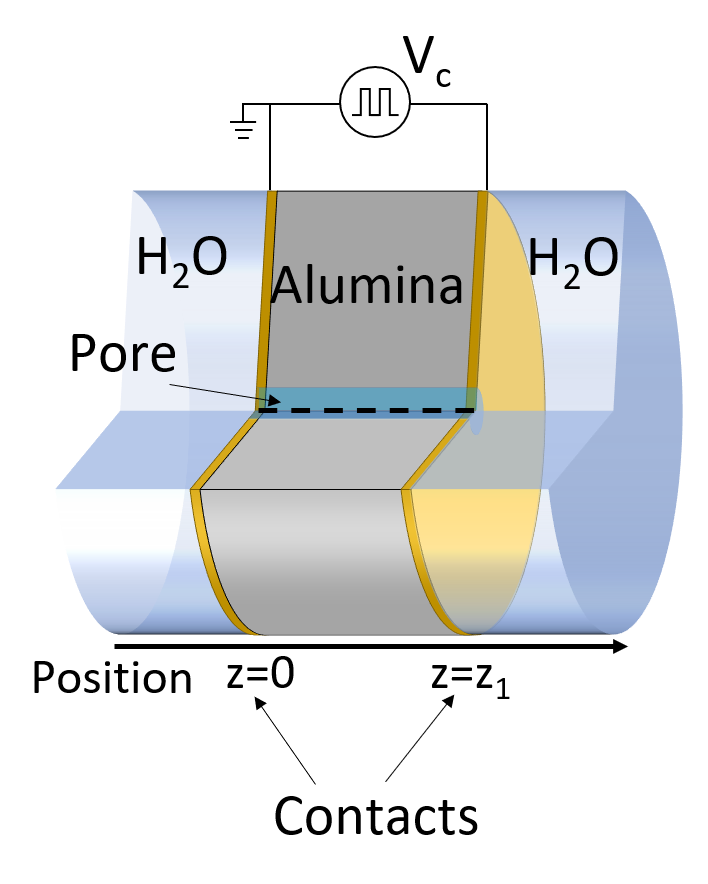
In this project we are developing a first-of-its-kind “all electric”, ion pump based on an electronic ratchet mechanism. Electronic ratchets utilize modulation in a spatially varying electric field to drive steady state current. Similar to peristaltic pumps, where the pump mechanism is not in direct contact with the pumped fluid, electronic ratchets induce net current with no direct charge transport between the power source and the pumped charge carriers. Thus, electronic ratchets can be used to pump ions in steady state with no electrochemical reactions between the power source and the pumped ions resulting in an “all electric” ion pump.
In this project we study both theoretical and experimental aspects of ratchet based ion pumping- from basic thermodynamics, through advanced finite elements device simulations, on to device fabrication and characterization and finally designing electrochemical system based on our ion pumps.
Collaborations:
Functional tomography of Solar Cells
Multi-dimensional mapping of charge transport and loss in solar cells
The next generation of solar energy conversion systems requires design and integration of new semiconductor materials. Detailed understanding of the opto-electronic properties of these materials and the driving forces and loss mechanisms that limit device performance is essential to the development of high efficiency systems. However, these materials and systems are difficult to model and only few experimental methods are available for direct characterization of dominant loss processes under relevant operating conditions.
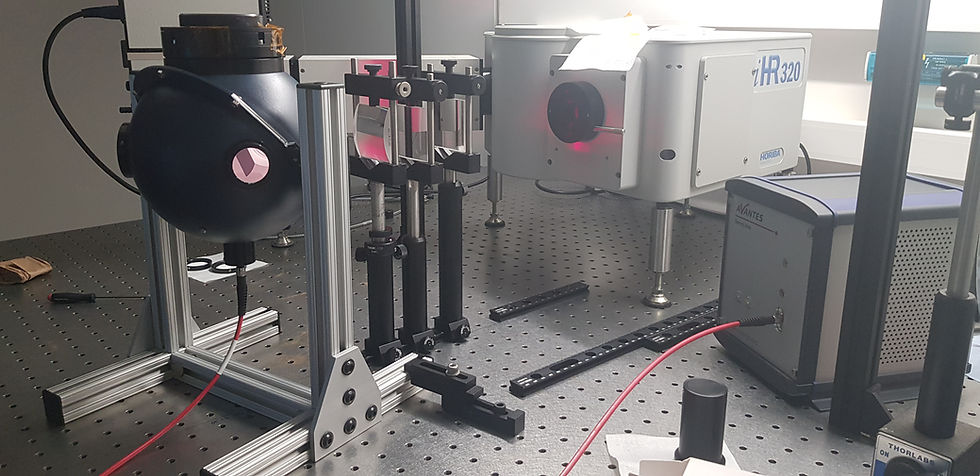
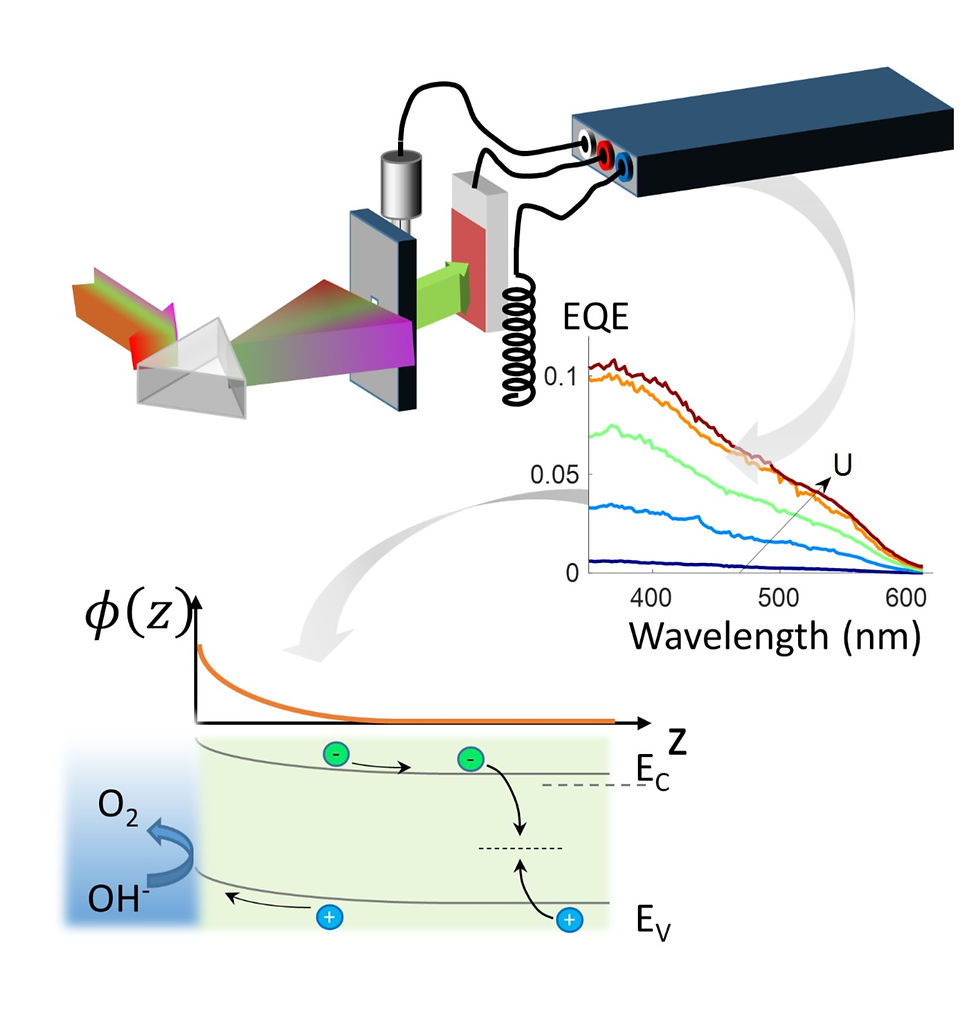

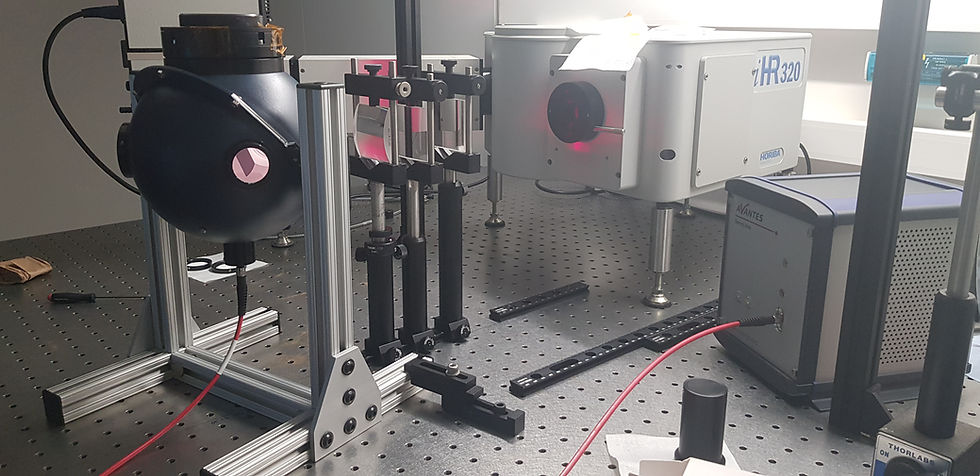
To this end, empirical extraction of the spatial collection efficiency (SCE) and the spatial external luminescence efficiency (SELE) are operando, analytical tools that provides a functional depth profile of the active regions in the device. By coupling external quantum efficiency (EQE) measurements and optical modeling, SCE extraction allows quantifying charge transport properties and loss mechanisms across the device depth profile under real operating conditions with very few assumptions. In a similar manner, combining optical modeling with photoluminescence quantum yield measurements allows extracting the SELE which can be used to quantify important device characteristics such as surface recombination velocities and map the competition between of radiative and non-radiative recombination processes.
Collaborations:
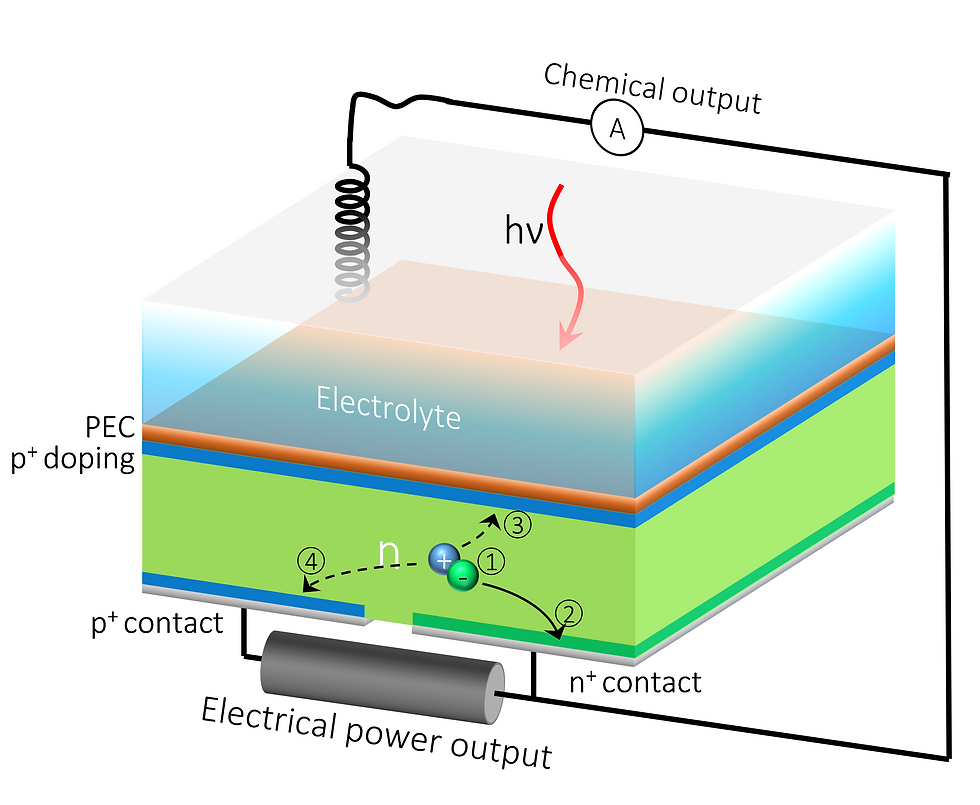
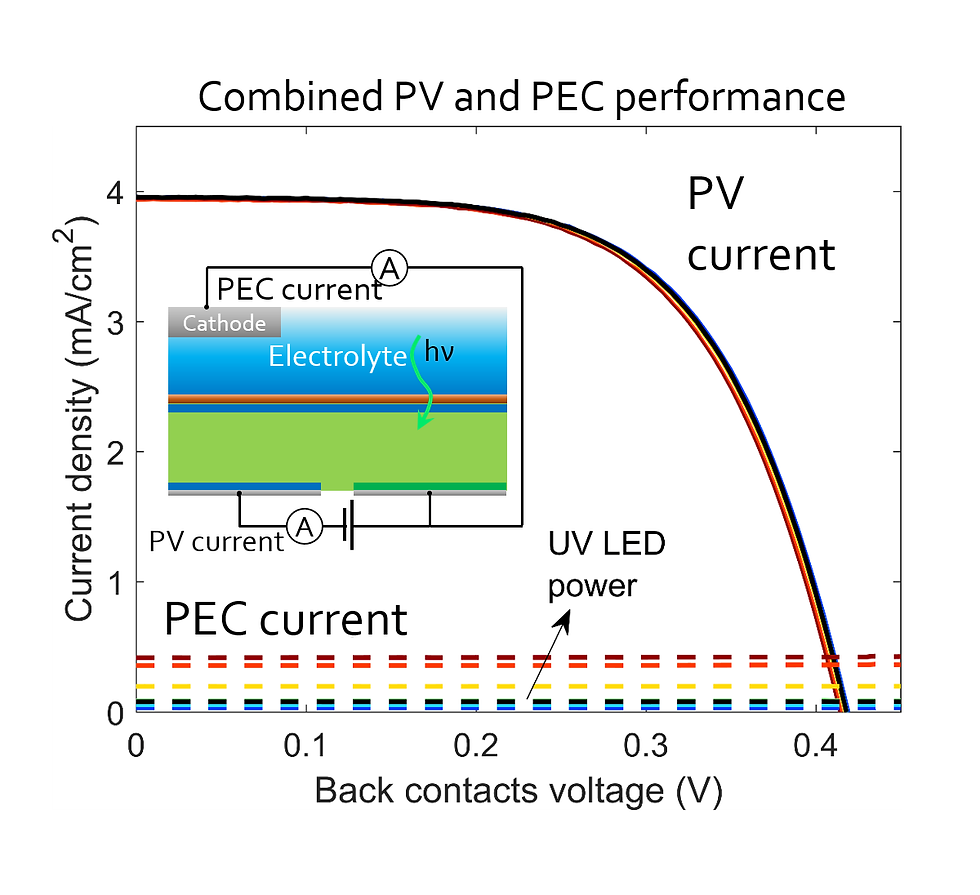
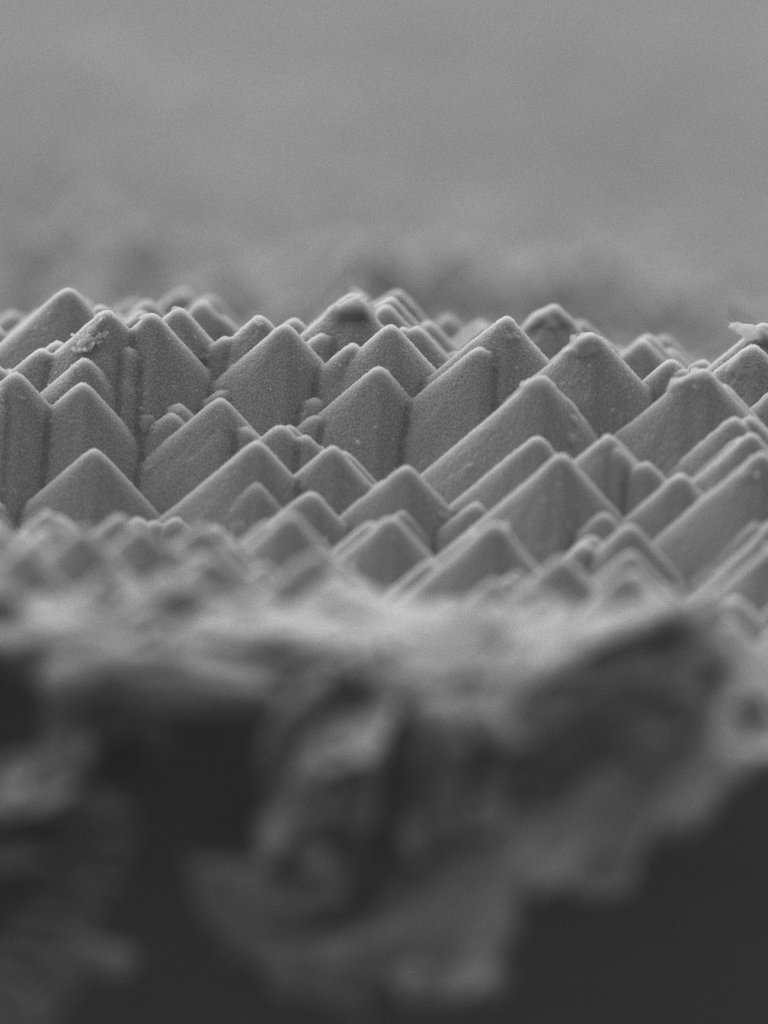
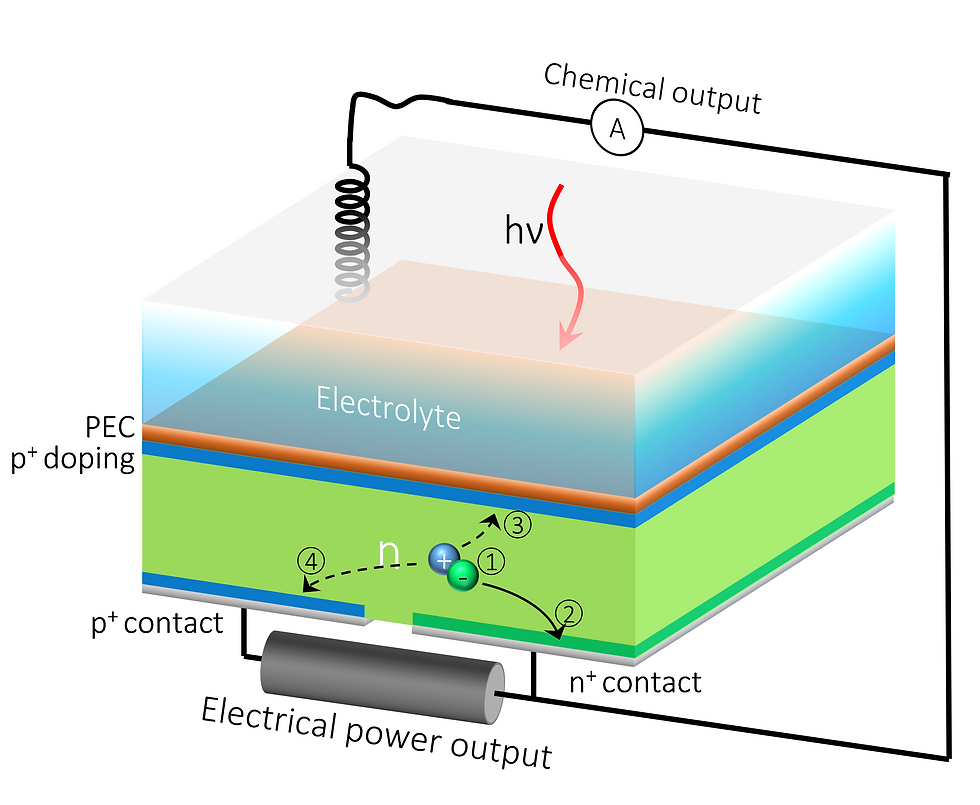
HPEV cells
Hybrid Photo-Electrochemical and Photo-Voltaic Cells
Over the years, considerable work was dedicated to solar powered water decontamination and hydrogen generation. However, in most cases the demonstrated devices were made of wide band gap absorbers which are inherently limited as solar energy powered devices. Higher performance can be obtained by stacking two or more light absorbers such that each absorbs a different part of the spectrum. However, multi junction photoelectrochemical (PEC) cells suffer from considerable losses due to imperfect electrical coupling between the layers.
​
To remove these coupling losses, we have introduced the Hybrid Photoelectrochemical and Photovoltaic (HPEV) cell- a dual junction PEC cell in which a second back contact allows extracting energy that is not consumed by the chemical reaction as electrical power. As a result, HPEV cells generate electricity at their maximum power point with hardly impeding hydrogen generation.
Although demonstrating a first of its kind semiconductor device capable of solar powered hydrogen production and electricity generation, the efficiency of the first prototypes was fairly low. In this project we aim to advance this technology significantly further, increasing its efficiency and adopting it for water decontamination photoelectrochemistry. By combining the HPEV architecture with water purification photoelectrochemistry we will fabricate cells that remove contaminations from water while generating hydrogen and utilize energy that is not consumed by the chemical reactions to generate electricity. Combining solar powered electricity generation with photoelectrochemistry may provide opportunities for solar water desalination as well. Thus, a successful realization of the proposed HPEV cells may provide all in one solutions for issues our society will confront in the coming years and are currently faced by much of the developing world.